In the grand scheme of life, DNA oligonucleotides are the unsung heroes, stitching together the intricate patterns of gene expression. These short synthetic DNA or RNA polymers, typically 15-25 nucleotides long, have revolutionized molecular biology, giving researchers unprecedented control over the building blocks of life. From their starring role in research labs to their groundbreaking applications in fields like mRNA vaccines and gene therapy, DNA oligonucleotides are the Swiss Army knives in our quest to understand and manipulate biological systems.
In this comprehensive guide, we'll dive deep into the captivating world of DNA oligonucleotides. We'll explore how they are made, their diverse uses in research and medicine, and the challenges we face in harnessing their full therapeutic potential. Whether you're a curious student, a seasoned researcher, or simply fascinated by the power of these tiny molecules, this article will provide you with a thorough understanding of DNA oligonucleotides and their far-reaching impact.
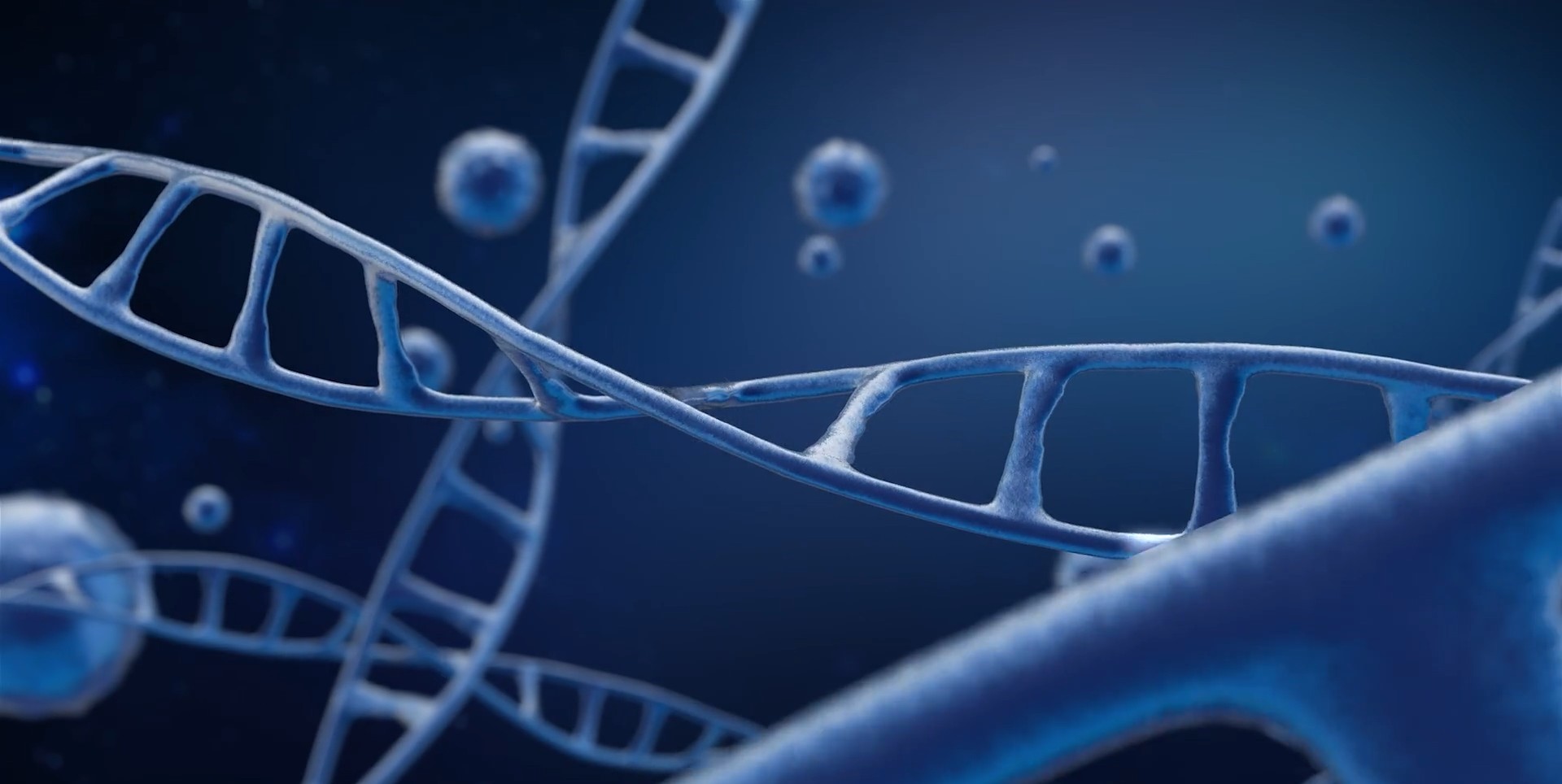
How DNA Oligonucleotides Are Made
The creation of DNA oligonucleotides is a marvel of modern science. These precise sequences are crafted in the lab using a process called solid-phase synthesis. This automated assembly line allows for the step-by-step addition of nucleotides in any desired order, with each base being added one at a time to a growing chain anchored to a solid support.
The process begins with the 3' end of the oligonucleotide attached to a solid support, usually a glass bead or a polystyrene resin. The nucleotides are then added sequentially in a 3' to 5' direction, with each addition involving several chemical steps:
-
Deprotection: The protecting group on the 5' end of the growing chain is removed, making it ready to react with the next nucleotide.
-
Coupling: The next nucleotide, which has a protective group on its 5' end and a reactive group on its 3' end, is added. The reactive group on the incoming nucleotide forms a bond with the 5' end of the growing chain.
-
Capping: Any unreacted 5' ends are "capped" to prevent them from reacting in subsequent steps, thus reducing errors in the final oligonucleotide sequence.
-
Oxidation: The newly formed phosphite bond is oxidized to a more stable phosphate bond.
These steps are repeated until the desired sequence is achieved. Finally, the completed oligonucleotide is cleaved from the solid support and purified to remove any leftover protecting groups and impurities.
The solid-phase synthesis process is highly efficient, allowing for the production of oligonucleotides up to about 200 bases in length with high accuracy and yield. The automated nature of the process means that many different oligonucleotide sequences can be synthesized in parallel, greatly accelerating research and application timelines.
The Diverse Uses of DNA Oligonucleotides
Once synthesized, DNA oligonucleotides are ready to be put to work in a wide variety of applications that span the realms of research, biotechnology, and medicine. Let's explore some of the key areas where these versatile molecules make their mark:
Gene Expression Modulation
One of the most powerful applications of DNA oligonucleotides is their ability to modulate gene expression. By designing oligonucleotides that are complementary to specific gene sequences, researchers can control whether a gene is turned on or off, or even edit its sequence.
Antisense oligonucleotides (ASOs) are one example of how this works. ASOs are designed to bind to mRNA, the intermediary between DNA and protein, and either prevent its translation into protein or trigger its degradation. This gene silencing effect can be harnessed for research purposes, such as studying the function of a particular gene, or for therapeutic applications, such as treating diseases caused by the overexpression of a specific gene.
Another approach is the use of small interfering RNAs (siRNAs), which are short double-stranded RNA oligonucleotides that can also silence genes via a process called RNA interference (RNAi). Like ASOs, siRNAs can be designed to target specific mRNAs for degradation, thus preventing the production of the corresponding protein.
Genome Editing
DNA oligonucleotides also play a crucial role in genome editing technologies like CRISPR-Cas9. In this system, a guide RNA (gRNA) is used to direct the Cas9 endonuclease to a specific location in the genome, where it makes a double-stranded break in the DNA. Researchers can then use DNA oligonucleotides as templates for the cell's own repair machinery to introduce specific changes at the cut site, such as correcting a disease-causing mutation or inserting a new gene.
This precision editing of the genome holds immense promise for treating genetic disorders, creating disease models for research, and even enhancing crops and livestock. The ability to make such targeted changes is a testament to the power and versatility of DNA oligonucleotides.
PCR and DNA Sequencing
In the realm of molecular biology research, DNA oligonucleotides are indispensable tools. They form the backbone of techniques like polymerase chain reaction (PCR) and DNA sequencing, which have revolutionized our understanding of genes and genomes.
In PCR, short DNA oligonucleotides called primers are used to selectively amplify a specific region of a DNA template. By designing primers that flank the region of interest, researchers can generate millions of copies of that sequence, enabling further analysis and manipulation. PCR has become a staple in labs worldwide, used for everything from diagnosing infections to studying gene expression.
DNA sequencing, the process of determining the precise order of nucleotides in a DNA molecule, also relies heavily on oligonucleotides. In the widely used Sanger sequencing method, DNA oligonucleotides serve as primers to initiate the sequencing reaction. Newer sequencing technologies, such as Illumina's sequencing by synthesis, use oligonucleotides attached to a solid surface to capture and sequence DNA fragments.
The ability to rapidly and accurately sequence DNA has opened up entire new fields of study, from genomics to personalized medicine. None of this would be possible without the precision and reliability of DNA oligonucleotides.
mRNA Vaccines
In recent years, DNA oligonucleotides have taken center stage in the development of a new class of vaccines: mRNA vaccines. These vaccines work by delivering genetic instructions, in the form of mRNA, to cells, directing them to produce specific proteins that stimulate an immune response.
The mRNA in these vaccines is synthesized via a process called in vitro transcription, which uses a DNA template containing the desired sequence. This DNA template is none other than a carefully designed DNA oligonucleotide.
The success of mRNA vaccines in the fight against COVID-19 has highlighted the immense potential of this technology. The speed at which these vaccines were developed and deployed is a testament to the power of synthetic oligonucleotides and our mastery over the language of genetics.
Challenges and Future Directions
Despite their vast potential, the use of DNA oligonucleotides in therapeutic applications is not without its challenges. One major hurdle is delivery: as large, negatively charged molecules, oligonucleotides cannot easily cross cell membranes to reach their intracellular targets. Researchers are working on developing sophisticated delivery systems, such as lipid nanoparticles or cell-penetrating peptides, to overcome this barrier.
Another challenge is stability. Oligonucleotides are susceptible to degradation by enzymes called nucleases, which can limit their half-life in the body. Chemical modifications, such as the addition of phosphorothioate bonds or 2'-O-methyl groups, can help increase their resistance to nuclease digestion.
Immunogenicity, or the ability to trigger an immune response, is also a concern with some oligonucleotide therapies. While this immune stimulation can be harnessed for applications like cancer immunotherapy, in other cases it may lead to unwanted side effects. Careful sequence design and chemical modifications can help mitigate these issues.
Despite these challenges, the field of DNA oligonucleotides is advancing at a rapid pace. Researchers are continually refining oligonucleotide design, synthesis, and delivery methods to improve their therapeutic efficacy and safety. The use of machine learning and artificial intelligence is also helping to accelerate the discovery and optimization of oligonucleotide drugs.
As our understanding of the complex language of genetics deepens, so too does the potential for DNA oligonucleotides to transform medicine. From correcting genetic defects to silencing disease-causing genes to programming our immune systems to fight cancer and infections, the possibilities are vast and exciting.
Conclusion
DNA oligonucleotides are a testament to our growing mastery over the building blocks of life. These short, synthetic sequences of DNA or RNA have proven to be incredibly versatile tools in the hands of researchers and clinicians alike. From their use in fundamental research techniques like PCR and sequencing to their application in cutting-edge therapies like gene editing and mRNA vaccines, oligonucleotides are at the forefront of modern molecular biology and medicine.
As we continue to unravel the mysteries of the genome and develop ever more sophisticated ways of manipulating it, DNA oligonucleotides will undoubtedly play a central role. The challenges of delivery, stability, and immunogenicity are not insurmountable, and the rapid pace of progress in this field is a cause for optimism.
In the grand tapestry of life, DNA oligonucleotides may be small, but their impact is immense. They are the threads that we use to weave our understanding of biology, to mend what is broken, and to create new possibilities for health and well-being. As we look to the future, it is clear that these unsung heroes will continue to shape the course of science and medicine in profound and exciting ways.